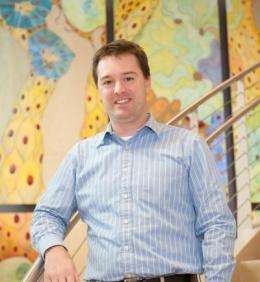
Stem cell technologies have been proposed for cell-based diagnostics and regenerative medicine therapies. However, being able to make stem cells efficiently develop into a desired cell type -- such as muscle, skin, blood vessels, bone or neurons -- limits the clinical potential of these technologies.
New research presented on June 16, 2011 at the annual meeting of the International Society for Stem Cell Research (ISSCR) shows that systematically controlling the local and global environments during stem cell development helps to effectively direct the process of differentiation. In the future, these findings could be used to develop manufacturing procedures for producing large quantities of stem cells for diagnostic and therapeutic applications. The research is sponsored by the National Science Foundation and the National Institutes of Health.
"Stem cells don't make any decisions in isolation; their decisions are spatially and temporally directed by biochemical and mechanical cues in their environment," said Todd McDevitt, director of the Stem Cell Engineering Center at Georgia Tech and an associate professor in the Wallace H. Coulter Department of Biomedical Engineering at Georgia Tech and Emory University. "We have designed systems that allow us to tightly control these properties during stem cell differentiation, but also give us the flexibility to introduce a new growth factor or shake the cells a little faster to see how changes like these affect the outcome."
These systems can also be used to compare the suitability of specific stem cell types for a particular use.
"We have developed several platforms that will allow us to conduct head-to-head studies with different kinds of stem cells to determine if one type of stem cell outperforms another type for a certain application," said McDevitt, who is also a Petit Faculty Fellow in the Institute for Bioengineering and Bioscience at Georgia.
Many laboratory growth methods allow stem cells to aggregate in three-dimensional clumps called "embryoid bodies" during differentiation. McDevitt and biomedical engineering graduate student Andres Bratt-Leal incorporated biomaterial particles directly within these aggregates during their formation. They introduced microparticles made of gelatin, poly(lactic-co-glycolic acid) (PLGA) or agarose and tested their impact on the assembly, intercellular communication and morphogenesis of the stem cell aggregates under different conditions by varying the microsphere-to-cell ratio and the size of the microspheres.
The researchers found that the presence of the biomaterials alone modulated embryoid body differentiation, but did not adversely affect cell viability. Compared to typical delivery methods, providing differentiation factors -- retinoic acid, bone morphogenetic protein 4 (BMP4) and vascular endothelial growth factor (VEGF) -- via microparticles induced changes in the gene and protein expression patterns of the aggregates.
By including tiny magnetic particles into the embryoid bodies during formation, the researchers also found they could use a magnet to spatially control the location of an aggregate and its assembly with other aggregates. The magnetic particles remained entrapped within the aggregates for the duration of the experiments but did not adversely affect cell viability or differentiation.
"With biomaterial and magnetic microparticles, we are beginning to be able to recreate the types of complex geometric patterns seen during early development, which require multiple cues at the same time and the ability to spatially and temporally control their local presentation," noted McDevitt.
While microparticles can be used to control differentiation by regulating the local environment, other methods exist to control differentiation through the global environment. Experiments by McDevitt and biomedical engineering graduate student Melissa Kinney have demonstrated that modulating hydrodynamic conditions can dictate the morphology of cell aggregate formation and control the expression of differentiated phenotypic cell markers.
"Because bioreactors typically impose hydrodynamic forces on cells to cultivate large volumes of cells at high density, our use of hydrodynamics to control cell fate decisions represents a novel, yet simple, principle that could be used in the future for the scalable efficient production of stem cells," added McDevitt.
Technologies capable of being directly integrated into bioprocessing systems will be the best choice for manufacturing large batches of stem cells, he noted. In the future, the development of multi-scale techniques that combine different levels of control -- both local and global -- to regulate stem cell differentiation may help the translation of stem cells into viable clinical therapies.
Provided by Georgia Institute of Technology
Post comments